The slime mold Physarum polycephalum is a single-celled organism that can convert to multiple forms and that displays surprising complexity in its behavior. In one of its forms, the single cell can become very large—more than 30 cm in diameter in a laboratory setting. In nature, Physarum polycephalum is found in multiple environments; however, the organism is most commonly found in cool, humid, dark places such as leaf litter and other organic debris in forests. It can be used to discuss multiple subjects, including life cycles, mitosis, meiosis, sexual and asexual reproduction, cytoplasmic streaming, chemotaxis, basic navigation, simple decision-making, mechanisms for survival in stressful environments, cell structure, and developmental biology.
Potential investigations
With a little care and attention, Physarum polycephalum is easy to grow in the classroom and is a good organism for students to use for independent investigation. Below are some ideas for student investigations, followed by background information on the organism.
- Have students construct a maze. In the plasmodial phase, the organism can become very large and forages a great deal. Have students construct a maze and test a Physarum plasmodium’s ability to find its way through it, or to connect a source of food at the beginning of the maze with a source of food at the end of the maze using the shortest path. Be aware that the plasmodium, depending upon the materials composing the maze and the ambient conditions, will sometimes cross the maze walls. Students may want to experiment with using different types of material to make the maze or using different things to coat the maze in order to avoid this.
- Investigate chemotaxis. Have students test the ability of different substances to attract or repel a Physarum plasmodium. Have students perform their test in multiples and use statistics to measure the significance of their observations.
- Have students explore the effects of temperature on the plasmodial form of Physarum.
- Have students examine the effect of the slime track that a Physarum plasmodium leaves behind when it exits an area on a plasmodium’s willingness to forage in that area.
- Under conditions of stress, Physarum polycephalum takes on a form referred to as a sclerotium. Have students explore what conditions cause a sclerotium to form. They could test the effect of desiccation, solutions of different metals, cold and hot temperatures, changes in pH, starvation in the dark, etc.
- Investigate the role of Physarum polycephalum as a decomposer.
Background on Physarum polycephalum
Many of the processes used by Physarum are similar to those found in more complex organisms. Thus, Physarum has been used as a model for studying many of the basic processes present in many organisms, including cell differentiation, cell cycle regulation, mitosis, meiosis, cytoskeletal rearrangement, and cytoplasmic streaming.
In addition, the ability of Physarum to navigate using relatively complex strategies to find food and to form networks between different sources of food has drawn the attention of people outside traditional areas of biological research, including those who study networks and artificial intelligence.
Physarum polycephalum life cycle
During its life cycle, Physarum polycephalum transitions into different forms, some of them haploid, others diploid, and some designed to protect the organism under harsh conditions. The organism’s transition from one form to another is most often triggered by the conditions of its environment, including the presence or absence of other Physarum.
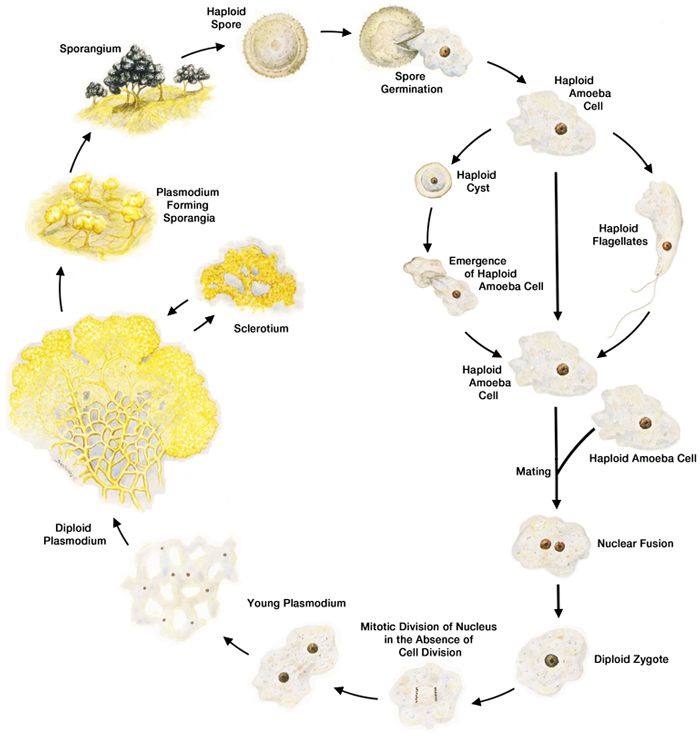
Plasmodial form
In the classroom, Physarum is most often observed in its diploid, plasmodial form. In its plasmodial form Physarum polycephalum exists as a single large cell containing multiple diploid nuclei that replicate their DNA and divide synchronously. These single cells are capable of becoming very large.
Sporulation
When a plasmodium is starved and then exposed to light, it will sporulate. In nature, the organism has been observed to sporulate after climbing out of leaf litter to where it gets exposed to light. These small, dark spores can survive for many years. Thus, the formation of spores is one way the organism ensures that it will survive harsh conditions until more favorable living conditions return. Sporulation is also the first step in sexual reproduction.
During early sporulation the organism forms fruiting bodies, which initially appear as bumps emerging from the surface of the plasmodium. These bumps develop into stalk-shaped structures that project from the plasmodium surface. When viewed under the microscope they are quite dramatic. A fully developed spore contains only a single haploid nucleus.
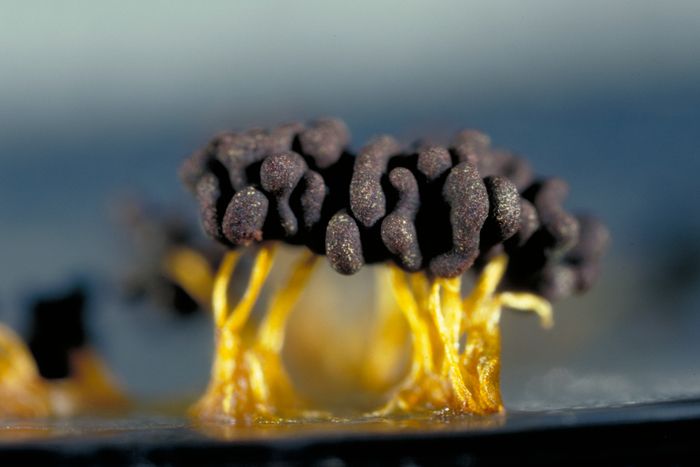
Amoebas and flagellates
When there is sufficient moisture, haploid, single nucleus amoebas emerge from the spores. In contrast to what occurs in the plasmodial form, mitosis in the amoeboid form is accompanied by cell division. Repeated division of the Physarum amoeba results in a colony of amoebas that are genetically the same.
The amoeba can change into 2 different forms, flagellates (cells with 2 flagella) or cysts. The transformations from amoeba to flagellate and from amoeba to cyst are both reversible. If an amoeba runs out of food or encounters other adverse conditions—for example, dryness—it forms a cyst with protective walls. When the conditions become more favorable again, an amoeboid cell reemerges from the cyst. Transformation to a flagellate occurs under wet conditions. Flagellates will transform back into amoebas under drier conditions. The amoeba’s ability to make these transformations allows the organism to survive a broader range of conditions than it would otherwise.
Sexual reproduction in slime mold
Genetic diversity is beneficial to the long-term survival of a species. As is true for most organisms, sexual reproduction is one of the mechanisms generating genetic diversity in Physarum polycephalum. The combining of genetic material from 2 different organisms occurs when 2 haploid amoebas fuse with each other to form a single organism with a single diploid nucleus. This single diploid cell then goes on to develop into a plasmodium. The mating of the 2 amoebas is controlled by several multi-allele mating loci.
Sclerotium
As discussed above, Physarum polycephalum when in the amoeboid form can respond to stressful conditions by forming a cyst. When in the plasmodial form, Physarum responds to starvation and light by sporulating. However, if the stressful conditions occur in the absence of light, the plasmodium will form a sclerotium, a collection of macrocysts (also called spherules) surrounded by a hard, dry protective layer. Sclerotia can form in response to a number of stimuli, including starvation, dryness, cold, low pH, and solutions with high osmotic pressure and exposure to some heavy metals. Depending upon the conditions, a sclerotium can be revived to the active form of the organism for months after it forms.
Foraging and streaming
Physarum in the plasmodial form uses phagocytosis to ingest its food, which consists of small particles of organic material, bacteria, and other microorganisms. It also secretes enzymes to break down materials, which are then absorbed by pinocytosis. As it forages, the plasmodial form of Physarum moves around using a slow flowing movement. The part of the organism that is at the forefront of foraging has a fanlike configuration. As the organism searches for and takes in food, the cell contents stream back and forth at approximately 60-second intervals through a network of vein-like looking tubes. A single vein can be up to 1 mm in diameter, and streaming can be easily observed using a stereomicroscope. Absorbed material gets distributed throughout the cell using this mechanism. The network of tubes is reorganized as the organism moves in search of food.
The periodic streaming is accomplished through the creation of hydraulic pressure gradients. Contraction of the actomyosin network within the plasmodium creates these pressure gradients. The actomyosin network is part of an extensive network of microfilaments that exists throughout the entire plasmodium. Multiple other proteins are associated with this network. As a group, these proteins are referred to as actin-binding proteins and play a role in carrying out the functions of the actomyosin network, including its reorganization.
Foraging strategies of Physarum
The strategies used by the plasmodial form of Physarum to find food are surprisingly complex. At a basic level, the organism senses food at a distance, detects when it moves closer to or farther away from the food, and adjusts its movement according to the input it receives. This is a simple example of chemotaxis. However, the organism has at least one additional strategy for optimizing how it searches an environment for food. When seeking food, the plasmodium avoids any areas it has explored until it has covered all unexplored areas. This behavior is thought to increase the efficiency of its foraging. This type of foraging strategy has long been observed and studied in more complex organisms with internal neurologic memory that can be used to remember where the organism has been. However, Physarum polycephalum is a unicellular organism without a neural network for remembering. In the absence of a neurological memory, the organism creates a type of external memory by leaving a slime trail in areas from which it withdraws. The organism then strongly avoids areas with a slime track until it has explored all areas without residual slime or unless a new food source is placed in the slime-covered area.
In addition, as part of its foraging behavior Physarum can form networks connecting multiple food sources. These networks are similar to those created by humans with respect to efficiency. For example, a research group interested in the cost, efficiency, and resilience of networks hypothesized that a biological organism such as Physarum polycephalum, which has had its network forming strategies honed by years of natural selection, may provide good inspiration for the creation of networks that optimally balance these 3 characteristics. The research group created a model of the Tokyo area for Physarum polycephalum by placing food sources at the places representing the locations of major cities along the Tokyo rail network. Geographical features that had constrained the building of rail lines were represented as illuminated areas that would similarly be avoided by network-forming Physarum. The network built by the Physarum placed in this representative model was very similar to the existing rail line network.
In addition, the organism can make relatively complex decisions regarding its diet. Plasmodia with access to patches of food, which varied with respect to the carbohydrate-to-protein ratio and in the concentrations of each, migrated to the patch that provided the optimal diet.
The Physarum polycephalum genome
Sequencing the 250 megabase genome of Physarum polycephalum has been difficult because it has many regions where a short, specific sequence of nucleotides repeats many times in a row and areas where a single type of nucleotide is repeated many times. These characteristics make sequencing challenging and make assembling a complete genome from the sequences obtained difficult. To supplement the data obtained from sequencing the genome, researchers created a transcriptome. A transcriptome is a collection of the sequences of the RNAs transcribed by a certain cell type. In this case the “cell type” is actually the complete organism. The researchers pooled transcriptome sequence data from multiple life cycle stages. Computer analysis of the data predicted the presence of 34,438 genes. Half of these predicted gene loci could be linked to a transcript. Analysis of this data and comparison to similar data from closely related and even not so closely related organisms has provided information regarding the evolutionary relationships between the different organisms. For example, 2 phytochrome genes found in the Physarum genome appear to code for proteins that are most similar to bacterial phytochrome proteins. They also show some similarity to plant phytochromes. Interestingly, other apparent photoreceptor genes in the Physarum genome show the greatest similarity to gene sequences in animals. More studies will need to be done to elucidate the connections suggested by this data. These types of studies may one day make it clear how Physarum polycephalum came to possess characteristics displayed in organisms that occupy very different categories.
References
Alim, K., G. Amselem, F. Peaudecerf, M. P. Brenner, and A. Pringle. 2013. Random network peristalsis in Physarum polycephalum organizes fluid flow across an individual. Proceedings of the National Academy of Sciences 110 (33):13306–13311.
Bailey, J. 1995. Plasmodium development in the myxomycete Physarum polycephalum:Genetic control and cellular events. Microbiology 141:2355–2365.
Bailey, J. 1997. Building a plasmodium: Development in the acellular slime mould Physarum polycephalum. BioEssays 19 (11):985–992.
Daniel, J. W., and H. P. Rusch. 1962. Niacin requirement for sporulation of Physarum polycephalum. Journal of Bacteriology 83:1244–1250.
Dussutour, A., T. Latty, M. Beekman, and S. J. Simpson. 2010. Amoeboid organism solves complex nutritional challenges. Proceedings of the National Academy of Sciences 107 (10):4607–4611.
Jump, J. A. 1954. Studies on sclerotization in Physarum polycephalum. American Journal of Botany 41 (7):561–567.
Kawano, S., T. Kuroiwa, and R. W. Anderson. 1987. A third multiallelic mating-type locus in Physarum polycephalum. Journal of General Microbiology 133:2539–2546.
Moriyama, Y., and S. Kawano. 2003. Rapid, selective digestion of mitochondrial DNA in accordance with the matA hierarchy of multiallelic mating types in the mitochondrial inheritance of Physarum polycephalum. Genetics 164:963–975.
Reid, C. R., T. Latty, A. Dussutour, and M. Beekman. 2012. Slime mold uses an externalized spatial “memory” to navigate in complex environments. Proceedings of the National Academy of Sciences 109 (43):17490–17494.
Reid, C. R., M. Beekman, T. Latty, and A. Dussutour. 2013. Amoeboid organism uses extracellular secretions to make smart foraging decisions. Behavioral Ecology 24 (4):812–818.
Schaap, P., et al. 2016. The Physarum polycephalum genome reveals extensive use of prokaryotic two-component and metazoan-type tyrosine kinase signaling. Genome Biology and Evolution 8 (1):109–125.
Stockem, W., and K. Brix. 1994. Analysis of microfilament organization and contractile activities in Physarum. International Review of Cytology 149:145–215.
Tero, A., S. Takagi, T. Saigusa, K. Ito, D. P. Bebber, M. D. Fricker, K. Yumiki, R. Kobayashi, and T. Nakagaki. 2010. Rules for biologically inspired adaptive network design. Science 237:439–442.
Youngman, P. J., R. W. Anderson, and C. E. Holt. 1981. Two multiallelic mating compatibility loci separately regulate zygote formation and zygote differentiation in the myxomycete Physarum polycephalum. Genetics 97:513–530.